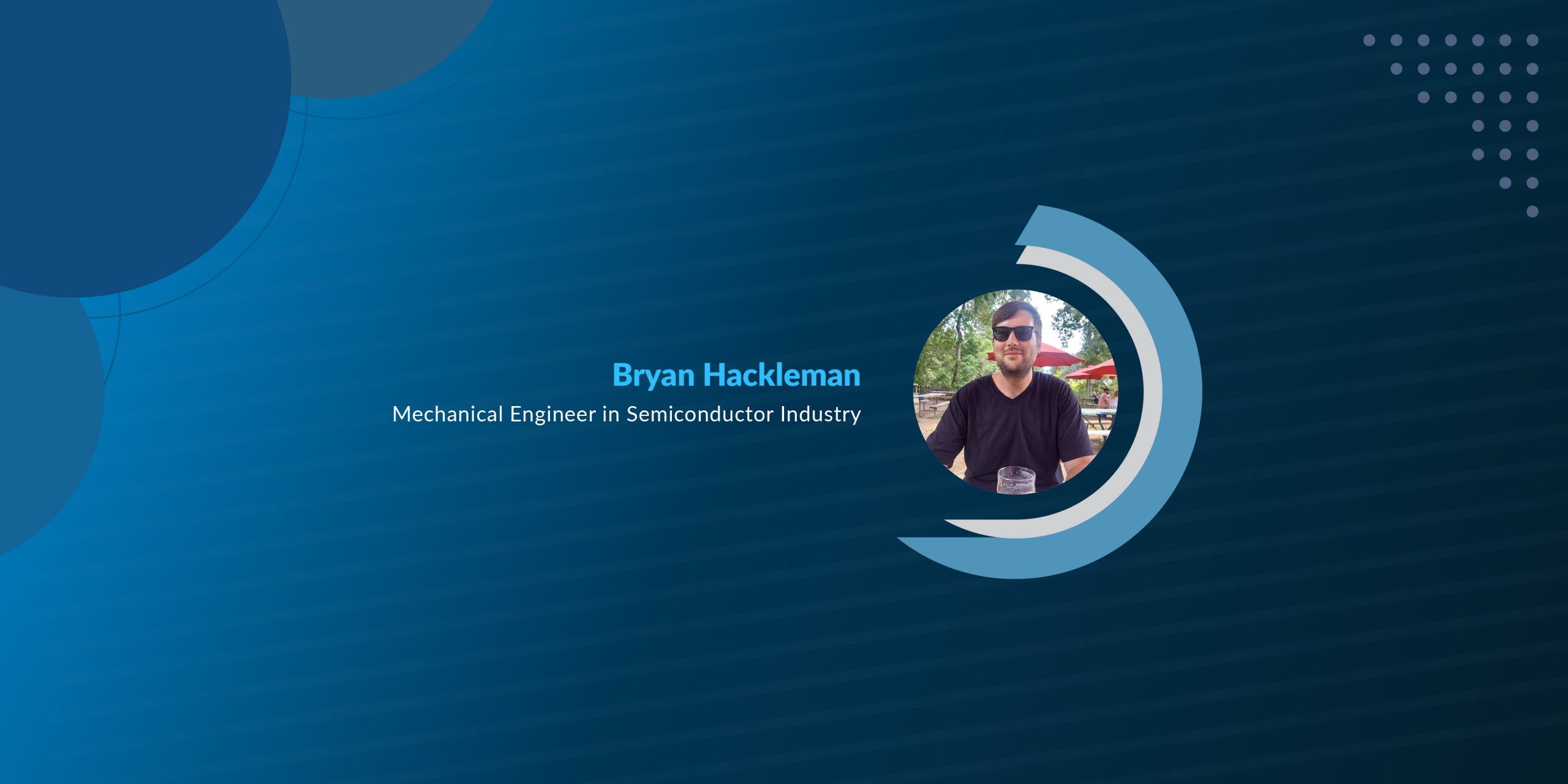
Mechanical engineering and robotics, two fields often seen as distinct, are deeply intertwined. Mechanical engineering principles form the backbone of robotic design, empowering machines with movement, strength, and versatility to accomplish complex tasks. From the precision of industrial arms to the fine-tuned dynamics of humanoid robots, mechanical engineering underpins every structural, functional, and kinetic aspect of robotics. As industries seek more efficient solutions and societies demand greater convenience, understanding the mechanical principles behind robotics reveals how this synergy drives innovation across sectors.
The Structural Framework: Ensuring Stability and Precision
One of mechanical engineering’s foundational contributions to robotics is developing a strong yet lightweight structural framework. Designing a robot requires a thorough understanding of materials, stress distribution, and load-bearing capacities. This framework, built upon mechanical engineering principles, supports the robot’s physical structure and protects its sensitive electronic components. The right choice of materials, typically involving metals like aluminum or advanced polymers, balances strength with the flexibility required for mobility.
The engineering of joints and connectors within a robotic structure plays a pivotal role in determining stability. Robotic arms, for instance, need to withstand and distribute varying forces while maintaining precision. For industrial robots, accuracy is critical, as they handle delicate assembly tasks or heavy-duty operations in manufacturing plants. Mechanical engineering principles such as torque calculations and stress analysis guide the design of these components, ensuring that the robot performs consistently and safely. By applying these fundamentals, engineers create robots that hold up under heavy use and achieve millimeter-level precision, which is essential in electronics assembly and medical device production.
Dynamics and Motion: Bringing Robots to Life
A robot’s motion is a key aspect of its functionality, and this is where dynamics—a branch of mechanical engineering—comes into play. Dynamics involves the study of forces and the resulting motion, and in robotics, this is applied to create smooth, coordinated movements. Actuators, motors, and gears are designed and calibrated based on dynamics principles, allowing robots to move with accuracy and control. Whether a robot is lifting, rotating, or gliding across surfaces, its motions must be predictable and repeatable to meet industrial and practical standards.
In addition to movement, the speed and acceleration of robotic actions are calculated through dynamics. Engineers must consider how quickly a robot can execute a movement without compromising its structural integrity. This is particularly important in high-speed assembly lines where robots must move parts rapidly yet carefully. For instance, calculating the angular velocity of a robotic joint enables engineers to design robots that can handle rapid motions without causing wear or excessive vibration, thereby extending their lifespan and efficiency.
Kinematics and Control Systems: Orchestrating Complex Tasks
While dynamics provides the blueprint for movement, kinematics governs the range and fluidity of motion. Kinematics is the study of motion without considering the forces that cause it. Mechanical engineers apply kinematic principles in robotics to determine how to control joint rotations, arm extensions, and other movements. Using kinematic equations, engineers calculate the ideal positions and angles for each joint in a robotic arm, enabling it to reach specific locations in space with precision.
Control systems play an integral role here, managing the robot’s real-time movement. These systems are based on feedback loops, which continuously adjust a robot’s actions to ensure it remains within predefined parameters. Mechanical engineers apply principles of control theory to design these feedback mechanisms, allowing robots to make real-time adjustments. For example, in medical robotics, a surgical robot relies on precise kinematic calculations and feedback control to perform delicate procedures with minimal human intervention. By adjusting its movements based on real-time input, the robot can compensate for slight shifts, maintaining accuracy essential to patient safety.
Energy Efficiency and Power Systems: Fueling Robotic Performance
Another critical area where mechanical engineering principles are applied is a robot’s energy requirements. Robots operate on limited power, and ensuring power is used efficiently is crucial for performance and sustainability. Mechanical engineers use principles of thermodynamics and energy conversion to design power-efficient robots. Engineers can reduce energy consumption without sacrificing functionality by optimizing the design of motors and actuators.
Battery technology and energy management are also key considerations. For autonomous robots, battery life dictates their operational range, and inefficient energy use can limit a robot’s productivity. Mechanical engineers employ strategies to enhance energy storage and conservation, allowing robots to operate for extended periods. This is particularly important for mobile robots or drones, which require a careful balance between power consumption and weight. Lightweight materials, efficient motors, and intelligent power distribution systems are applied to maximize energy use, giving robots the endurance needed for applications like logistics, surveillance, and search-and-rescue missions.
Safety and Reliability: Building Robots That Last
Safety and reliability are essential for any robot, especially those interacting with humans. Mechanical engineers apply rigorous safety standards when designing robots, incorporating factors such as stress limits, fail-safe mechanisms, and redundancy systems. Safety is paramount in industrial settings where robots perform tasks alongside human workers. Mechanical engineers must ensure these robots can detect and respond to potential hazards, often incorporating features like force sensors and emergency stop systems.
Reliability is also achieved through durability testing and simulation, both in mechanical engineering. Engineers use simulations to predict how robots behave under various conditions, identifying potential failure points and addressing them before deployment. This proactive approach is particularly important in applications where robot failure could have severe consequences, such as in aerospace or medical fields. By understanding and addressing these risks, mechanical engineers build robots that are safe, dependable, and able to withstand demanding environments.
Innovations in Mechanical Engineering Driving the Future of Robotics
As mechanical engineering principles evolve, so does the potential for robotic advancements. The development of new materials, such as carbon fiber composites and lightweight alloys, allows for the creation of stronger yet lighter robots. Advances in miniaturization mean that robotic components, from actuators to control systems, are becoming smaller and more efficient, allowing robots to perform tasks with even greater precision.
Robots are also benefiting from the integration of artificial intelligence (AI), which relies heavily on real-time data and control algorithms developed through mechanical engineering principles. AI-driven robots must process data and adjust movements instantaneously, which requires highly responsive control systems rooted in engineering principles. This blending of mechanical engineering with AI enables the creation of autonomous robots capable of complex decision-making, paving the way for applications in autonomous vehicles, smart manufacturing, and beyond.
Mechanical engineering is also at the forefront of sustainable robotics, emphasizing reducing the environmental impact of robotics manufacturing and operation. Engineers are exploring ways to make robots from recyclable materials, reduce waste in manufacturing, and design robots that can be easily repaired and upgraded. These innovations align with broader societal sustainability goals, ensuring that robotics will continue to evolve responsibly.
Mechanical engineering principles are the cornerstone for robotics, infusing robots with the stability, motion, efficiency, and safety they need to perform effectively. From ensuring precise movement to conserving energy and enhancing reliability, mechanical engineering shapes every aspect of robotic design and operation. As technological advances emerge, the role of mechanical engineering in robotics will expand, driving the next wave of innovation. These advancements will make robots more capable and efficient and empower them to serve society in previously unimaginable ways, redefining the boundaries of what robots can achieve.