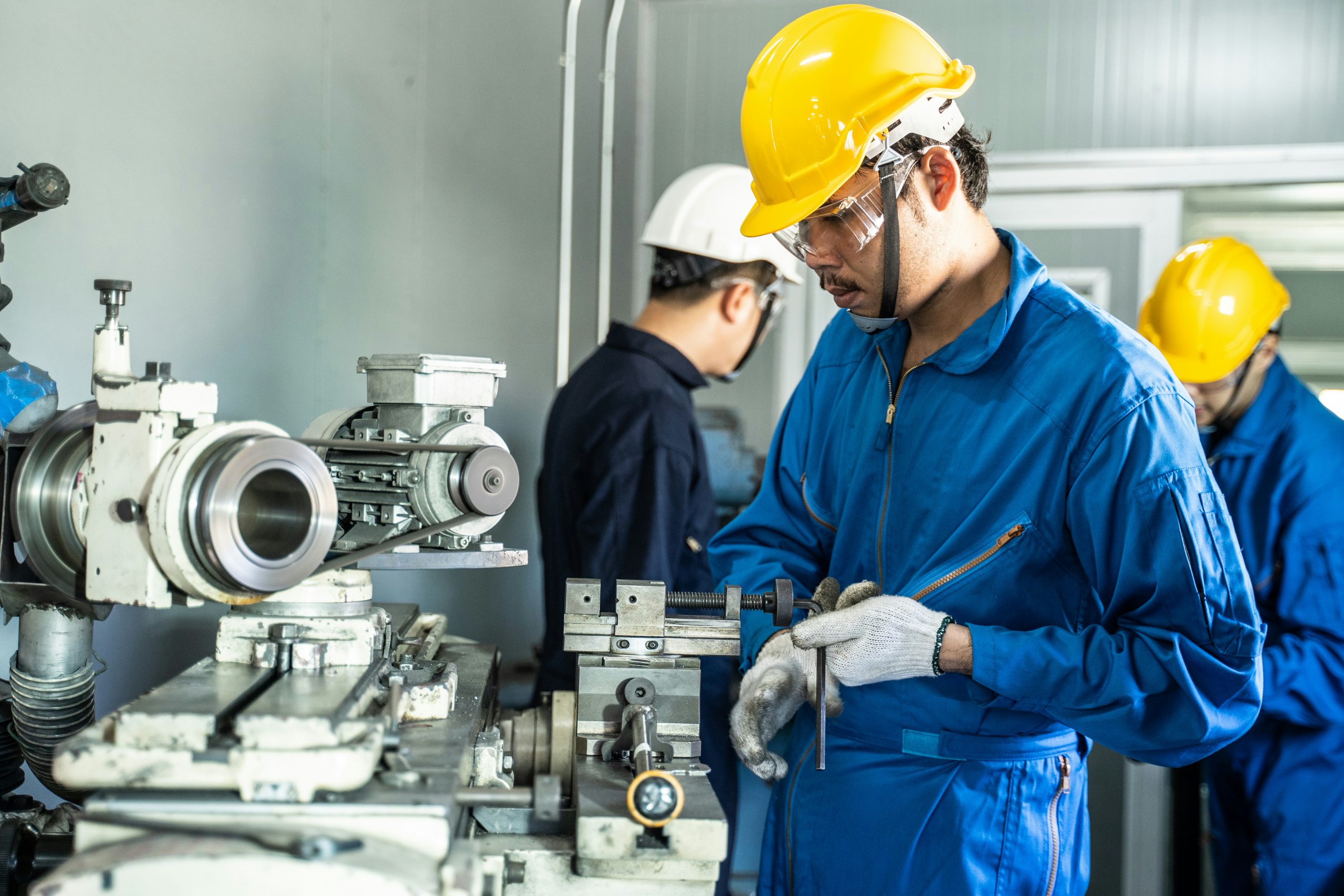
Mechanical engineering is one of the broadest and most fundamental branches of engineering, playing a crucial role in industries ranging from aerospace to automotive. At the heart of mechanical engineering are core principles guiding engineers in designing, analyzing, and improving mechanical systems. These principles form the foundation of problem-solving and decision-making in the field. Understanding them is essential for every aspiring engineer. Below, we explore these key principles.
Thermodynamics: The Science of Energy Transformation
Thermodynamics is a cornerstone principle of mechanical engineering. It studies energy and its transformations, mainly how heat energy can be converted into work. Engineers use thermodynamics to design efficient systems, such as engines, refrigerators, and air conditioning units. By applying the laws of thermodynamics, engineers can predict the behavior of fluids and gases within these systems and optimize their efficiency.
In addition to its direct applications in machinery, thermodynamics also plays a pivotal role in understanding the performance limits of energy conversion devices. For example, the second law of thermodynamics introduces the concept of entropy, which helps engineers identify system inefficiencies. Understanding these inefficiencies allows for system design and energy consumption improvements, which is essential in the modern world, where sustainability and energy conservation are increasingly prioritized.
Mechanics: The Study of Forces and Motion
Mechanics is another fundamental principle that every mechanical engineer should master. This branch of physics studies the behavior of forces and motion on solid bodies. Mechanics is broken down into several key areas: static mechanics, dynamic mechanics, and material mechanics. Static mechanics focuses on objects at rest, while dynamic mechanics deals with forces and motion in objects in motion. Material mechanics investigates the strength and behavior of materials under different loads.
Understanding the mechanic’s fundamentals enables engineers to design structures and machines that can withstand the forces they encounter. For instance, an engineer working on the design of a bridge must consider static and dynamic loads, such as vehicle weight and wind pressure. By applying principles from mechanics, engineers ensure that the bridge can withstand these forces without collapsing.
Material Science: The Study of Materials’ Properties
In mechanical engineering, selecting the right material for a design is crucial for ensuring durability and functionality. Material science explores the properties of different materials—metals, polymers, ceramics, and composites—and how these materials respond to stress, heat, and environmental factors. The properties of materials, such as strength, elasticity, hardness, and thermal conductivity, must be understood to choose the best material for a given application.
An in-depth knowledge of material science allows engineers to select materials that meet mechanical requirements and factor in environmental conditions such as temperature, humidity, and corrosive elements. For instance, aerospace engineers must choose materials with high strength-to-weight ratios, while marine engineers select materials resistant to corrosion. This principle is essential to creating reliable and sustainable systems and products.
Fluid Mechanics: Understanding Fluids in Motion
Fluid mechanics is a fundamental branch of mechanical engineering that focuses on the behavior of liquids and gases. It is essential for designing fluid flow systems, such as pumps, pipelines, and ventilation systems. Fluid mechanics consists of understanding concepts like fluid statics (the study of fluids at rest) and fluid dynamics (the study of fluids in motion). Engineers use principles from fluid mechanics to predict how fluids will behave in different systems and optimize the design for better performance.
Moreover, fluid mechanics is critical when considering the interaction between fluids and solid boundaries. For example, a car’s aerodynamics design involves optimizing the airflow around the vehicle to reduce drag and improve fuel efficiency. In industrial applications, understanding how fluids behave under various pressure conditions ensures the safe and efficient operation of systems such as power plants and chemical reactors.
Control Systems: Managing and Directing Mechanical Systems
Control systems in mechanical engineering refer to using devices to regulate and manage mechanical systems. This principle ensures that machines, robots, and automated systems operate as intended. Engineers apply control theory to design systems that respond predictably to inputs and disturbances, adjusting to maintain desired output values. Feedback loops are often used in these systems to ensure accuracy and efficiency.
For instance, a thermostat is a simple control system that maintains the desired temperature in a room. The thermostat measures the room’s temperature, compares it to the set value, and activates the heating or cooling system to maintain the set temperature. More complex control systems, such as those in manufacturing processes or aircraft, require advanced algorithms and sensors to manage the system’s performance. Thus, a firm grasp of control systems is essential for engineers working in automation and robotics.
Kinematics and Dynamics: The Movement of Parts and Systems
Kinematics and dynamics are crucial principles in understanding the motion of mechanical components and systems. These two areas of mechanics are vital in fields such as robotics, vehicle design, and manufacturing processes, where parts must move precisely and controlled.
For example, when designing a robot arm, engineers must understand the kinematics to determine how each joint moves in space. Dynamics comes into play when calculating the forces required to move those joints smoothly and efficiently. Both fields are indispensable when designing machines that need to perform complex tasks with high precision and speed.
Heat Transfer: The Movement of Thermal Energy
Heat transfer is the process by which thermal energy moves from one body or material to another. This principle is crucial for many mechanical engineering applications, especially in temperature regulation systems like heat exchangers, cooling systems, and thermal insulation. Engineers apply the three primary methods of heat transfer—conduction, convection, and radiation—to design systems that effectively control temperature.
For instance, engineers must calculate how heat will flow between fluids in contact when designing a heat exchanger. The goal is to optimize heat transfer to achieve the desired temperature changes while minimizing energy losses. Heat transfer is also vital in designing electronic devices, where managing the temperature is essential to prevent overheating and ensure longevity. Thus, understanding heat transfer is integral to creating efficient, reliable mechanical systems.
The core principles of mechanical engineering provide the foundation for creating innovative solutions in various industries. Each area contributes to the overall functionality and efficiency of mechanical designs, from thermodynamics and fluid mechanics to material science and control systems. For engineers, mastering these principles is about understanding and applying theoretical concepts to solve real-world challenges. As technology evolves, a firm grasp of these fundamental principles will remain essential for developing the next generation of mechanical systems and devices.